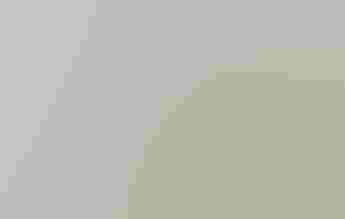
Henrik Mouritsen was stumped. It was supposed to be a simple experiment, just the latest iteration in a classic series of tests, something two generations of ornithologists had managed to pull off before him. And it was the very foundation of his research program—the kind of thing a junior faculty member had to get right. But for some reason, he couldn’t pull it off.
The idea was beautiful in its simplicity: Put captive songbirds—European Robins, in this case—in cages closed off from their surroundings, so the birds are unable to see sun, moon, or stars, and then record the direction they try to travel during the spring and fall migration seasons. Research dating back as far as the 1960s had shown that migratory birds kept in such conditions exhibit the jittery behavior known as Zugunruhe, German biologists’ parlance for “migratory restlessness.” Earlier studies had also revealed that birds have a clear directional preference. If a species breeds in the Northern Hemisphere, it inclines north in spring, south in fall, steered by the earth’s magnetic field. This experiment had been widely tested and accepted.
Then along came Mouritsen, an energetic Dane who holds on hard to his enthusiasms, which include nature photography, collecting Danish and Haitian stamps and Stone Age tools—and ornithology. Working in his lab at the University of Oldenburg, in a small city of 160,000 in the flatlands of northern Germany, he was hell-bent on finding out what parts of their brain songbirds use as they make navigational decisions. Yet by the mid-2000s, three years into his research, he was still struggling with Step 1—confirming the birds make those decisions in the first place. Mouritsen’s robins were exhibiting full-blown Zugunruhe in both spring and fall, but their movements showed no clear bearing. Something was screwing with their innate navigational sense, a built-in, fundamental element of their birdhood that has been observed in species as disparate as sparrows and chickens. It was as if a dog were to lack, for no apparent reason, its sense of smell.
“The experiments just didn’t work,” Mouritsen says. “I thought this was very strange, because that should have been the least of my experimental challenges. I tried adjusting all sorts of things, but nothing helped.”
Finally he had a laboratory conversation with Nils-Lasse Schneider, a colleague who studied the electrical signals given off by nerve cells. Schneider suggested that Mouritsen install electrically grounded metal panels that would shield the robins from certain wavelengths of electromagnetic radiation. It seemed like a shot in the dark. But then, why not, thought Mouritsen, worried that his ambitious research plan was about to implode on the runway.
Miraculously, the shielding worked. Mouritsen couldn’t believe it. It was as if a switch had been flipped on in the birds’ brains. Suddenly the robins behaved just as expected, sensing the magnetic field and clearly documenting with their footprints on paper their desire to move north or south, depending on the season. Mouritsen could go on with his research project, eventually locating a key area of the brain that appears to be vital for navigation and proving that it’s connected to visual processing—which suggests that birds need some light to effectively operate their internal compass.
That finding was enough to get Mouritsen a promotion to full professor well before his 40th birthday, as well as a prestigious German prize for neuroscience. But his initial failure nagged at him. Why had the robins been so mixed up? Could the cause really have been some unseen electromagnetic radiation?
There has been plenty of anxiety (though no certain scientific proof) that cellphones and high-voltage transmission lines might cause brain tumors. Mouritsen didn’t want to feed what he viewed as unproven and easily hyped concerns. But he did suspect that it might be some sort of electromagnetic radiation that interfered with the robins’ reading of the background magnetic field. He realized that his experimental hiccup might be a key clue to understanding how birds navigate.
As recently as the 19th century, many renowned naturalists were so bowled over by the mysterious phenomenon of migration that they speculated some birds spent the winter burrowed in the mud, or traveled all the way to the moon, or became different species with the change in seasons. Since then, extensive observation, increased knowledge of global migration patterns, and banding programs have helped ornithologists understand how daytime migrants, such as raptors and geese, travel by spotting landmarks, or by following coasts, ridgelines, and networks of thermals, where hot air rises. But the mystery of how tiny songbirds are able to find their way in the dark—flying at night helps them avoid predators—took much longer to unfold.
The turning point came in the 1940s and ’50s when a couple of German biologists, Gustav Kramer and Franz Sauer, pioneered the modern understanding of migratory navigation. Kramer caged songbirds, then noted that they would flutter in a particular direction when they felt the tug of Zugunruhe. Like the peripatetic hero of some country song, they moved with bills agape, wings quivering, the very picture of restlessness. Sauer came up with the idea of building glass-bottomed cages so that, lying underneath, he could closely watch what direction songbirds tended to move in on early autumn evenings. But the technique was tedious—and a prescription for backaches.
Then, in the early ’60s, a young University of Michigan graduate student named Stephen Emlen came up with a better idea: a circular cage centered on a saucer-sized inkpad. Around it he formed a sloping funnel of blotter paper. When he placed captive Indigo Buntings inside the cage, they inked their own feet—and then left at least part of the story of migration written on the blotter paper. (These days researchers tend to use thermal paper instead in what are still called Emlen funnels.)
“It was a way of quantifying how much a bird wants to jump to a particular direction,” says Emlen, now a professor emeritus at Cornell. “It was a simple technique—a way to clean out the ‘noise’ from our observations of migratory behavior.”
By conducting further tests in a planetarium, where he could obscure particular constellations or even whole tracts of the sky, Emlen was able to hone in on what his birds were looking at. The buntings, he soon discovered, were tuned to the rotation of the entire Northern Hemisphere sky around the North Star. When Emlen shifted the night sky, the buntings always reoriented themselves to move in the direction of the new north he had created; in autumn their tracks ran in the exact opposite direction.
That seemed logical to Emlen, since it’s very difficult to get accurate directional information from individual stars or constellations, all of which move during the course of a night and a season, and also appear at different positions to a migrating bird changing latitude. The buntings, he figured, were learning the same basic directional lessons that human navigators use. “A star by itself,” he concluded, “doesn’t say anything. But the birds do learn to recognize patterns. They do it the same way a Boy Scout learns to find the Big Dipper and the North Star—by getting organized movement from the pattern of stars.”
The big question was, how do migrants traveling on overcast nights stay oriented without visual cues? As Emlen did his work with buntings, a young German biologist named Wolfgang Wiltschko was probing the unseen. He placed captive European Robins into a solid steel cage in the basement of the University of Frankfurt’s zoological institute. Inside, the birds were shielded from the earth’s magnetic field. They were confused, and showed no particular directional impulse when it came time to migrate.
Then Wiltschko placed two pairs of large magnetic generators known as Helmholtz coils—physicists use them to experiment with electric fields—outside the cage. It was springtime, but he flipped the polarity so that the magnetic field lines that naturally direct north pointed to the southeast instead. It was a watershed moment. The Zugunruhe movements of his robins were clear: They wanted to travel southeast. He had found that, lacking all visual cues, the robins could orient themselves by sensing the earth’s magnetic field. Other biologists, though, were dubious.
“People really doubted this at first,” says Roswitha Wiltschko, Wolfgang’s wife and frequent research collaborator (both Wiltschkos are now retired from the University of Frankfurt, where they worked their entire careers). “They didn’t believe that a bird could navigate without celestial cues. To many people it seemed almost eerie that birds could do something that we cannot.”
Many subsequent studies, though, have showed that Wiltschko was right, and that in fact organisms of many species use the earth’s magnetic field to orient themselves. Bees, for example, have tiny receptors in their digestive systems that can tell them whether they are lined up with the magnetic field. Cattle and deer stand along a north-south axis more often than chance would explain. Researchers are currently investigating hints that seals may use magnetic cues to locate vital breathing holes in Antarctic ice. And in research that sounds as if it comes from the pages of The Onion, dogs exhibit a preference for defecating in line with the north-south axis.
Birds, it is now clear, have what amounts to a compass needle. They sense the magnetic lines running from each pole, which allows them to distinguish how north and south differ from all other directions. However, they can’t distinguish between north and south; they need further cues to know which direction to head. Think of a hiker arriving on the bank of a frozen river, unsure which way is downstream.
There’s ample evidence, too, of a strong interplay between the magnetic sense and the positions of the sun and stars: Birds use one set of directional cues to calibrate the others.
“There are redundant systems that give different types of info,” Emlen says—the stars, the sun, the magnetic field, the landscape, wind and weather. “The cues change in importance. Whatever a bird does, it’s going to have to have a good way of switching until it can pick up some new information.”
Further clues about how birds use multiple signs came in the mid-1960s from a talented electrical engineer named Bill Cochran, who worked for the Illinois Natural History Survey. After crafting tiny radio transmitters light enough to be attached to migrating songbirds, he began spending spring nights tracking night-flying thrushes by driving through the midwestern landscape—a challenging task. “In rural Illinois, most of the roads go straight north and south,” he recalls. “You were really humping it to keep up if the bird was going northwest or northeast. But if it weren’t for cops and speed limits, we could have followed them [all night].”
Cochran noticed a pattern in the thrushes’ movements: They would take off a little past sunset, and travel on a steady heading until roosting again in some patch of trees. By the mid-1970s he was confident they were using their magnetic sense to follow a straight line—and using the sunset direction to calibrate their built-in system by showing which way was more or less north and which was south.
Cochran also became an indispensable bridge from one generation to the next. He retired in 1992. But six years later he met another ambitious German biologist named Martin Wikelski, who was then working as a junior faculty member at the University of Illinois. Would Cochran, Wikelski wondered, show him and another colleague how he’d built his transmitters and tracked the thrushes?
He would. And so Cochran again found himself chasing birds through the Illinois dusk with a radio receiver, only this time with Wikelski and the colleague—who happened to be a young Henrik Mouritsen.
Wikelski now directs the Max Plank Institute for Ornithology in Radolfzell, in southern Germany, and he’s building on Cochran’s work in a big way. Next year a Roscosmos rocket is scheduled to lift off carrying four radio antennas that will be mounted on the International Space Station. Together they will constitute the central technology in what organizers call Project ICARUS—an effort to radio-track hundreds of species of animals at the same time from a vantage point that won’t be obscured. The process of miniaturization that Cochran jump-started in the 1960s has gone so far that Wikel-ski thinks even dragonflies will eventually be tracked from space.
“Emlen’s funnel system was a fantastic tool to create hypotheses that we can now test in the field,” he says. “But now we have more capacity to track animals than we have people to track them. This is the bare beginning. We need to have a global understanding about how animals migrate.”
Still, no one has been able to clarify just how birds sense the magnetic field. Some biologists, including the Wiltschkos, have stated that the magnetic sense is a visual faculty activated by exposure to certain wavelengths of light. Others have published studies suggesting that the stimulus isn’t visual at all, but is connected to magnetically sensitive minerals present in bird beaks. “I would put the word ‘seeing’ in quote marks in talking about this,” says Roswitha Wiltschko. “We really have no idea how they perceive what they do.”
Henrik Mouritsen’s experimental glitch may help scientists move one step closer to solving this puzzle. After he was able to get his research on track, he began a long series of studies. His students thought they were simply testing which brain areas are stimulated when a bird is feeling Zugunruhe. They were—but they were also testing something else. Mouritsen had designed an underlying study to look at the connection between electromagnetic radiation and the robins’ ability to distinguish direction. To do so, he set up paired sets of cages whose shielding equipment could be connected and disconnected—which he or a colleague would do in secret. To reduce the risk of bias about the effects of electromagnetic radiation, he didn’t tell his student researchers what he was up to. “I am absolutely meticulous in protecting against wishful thinking in the students,” he says.
After a couple of years, a pattern emerged. Robins kept on the university campus couldn’t orient using the earth’s magnetic field unless their cages were shielded with metal panels that block certain electromagnetic wavelengths. Robins at a different location, a mile outside the city limits, however, had no trouble. Furthermore, Mouritsen could create confusion in captive robins by placing them in shielded cages, then introducing wavelengths of electromagnetic radiation that were similar to those that had been blocked.
The implication was clear: Manmade electromagnetic waves present in the city but absent in a rural setting were disrupting the birds’ ability to sense the magnetic field, a major finding that landed his team’s research on the cover of the prestigious journal Nature last May.
Mouritsen is careful to provide caveats. The origin of the radiation was not a single source, and the frequencies at play were specifically not produced by cellphone or high-voltage transmission lines. Rather, the radiation was the inescapable background hum of the city—“electro-smog,” as some people call it. “It’s the sum total of everything you run on electricity,” he says. “It can come from anything that runs by current.” He hasn’t yet figured out a way to test whether there’s a threshold at which the radiation makes things go awry.
Studies show that urban buildings and communication towers kill hundreds of thousands of night-migrating birds each year, especially in overcast conditions. But more research is needed to tell how electromagnetic noise plays a role in those collisions, and how birds might cope if they lose access to one of their navigation tools. Mouritsen theorizes that they might simply switch to another means of navigation. “Their backup mechanisms would help them deal with this problem,” he says, but that, too, remains one of the important questions to be answered as we enter the dawn of a new frontier in ornithology, infinitely expanding what we know—and think we know—about birds and their journeys through the dark.
Peter Friederici is the author of Nature’s Restoration: People and Places on the Frontlines of Conservation. He teaches journalism at Northern Arizona University.